Nature Photonics Cover Story: A “Photon Decelerator” for Single Cycle Tunable Infra Pulses
Recently, a research article from Prof. Wei Lu’s group at Tsinghua University entitled "Relativistic, Single-cycle Tunable Infrared Pulses Generated from a Tailored Plasma Density Structure" has been published in the prestigious journal Nature Photonics, and chosen as the cover story for the August issue (Fig. 1). In this article, a novel scheme for tunable relativistic single-cycle infrared pulses is proposed, where a specially tailored plasma density structure is utilized to function as a “photon decelerator” (a nonlinear optical frequency converter) to efficiently convert a near-infrared laser with a wavelength of 0.8-1 μm to a relativistic near-single-cycle infrared pulse with tunable wavelength in the range of 5-14 μm. The referees of Nature Photonic highly appreciated the novelty and feasibility of this idea and expect it to be verified soon in future experiments. This new single cycle infrared source will definitely fill the long-standing gap in intense ultra-short infrared lasers in the 5-14 μm range, opening up new opportunities for relativistic infrared nonlinear optics, and for its application in attosecond science, ultrafast chemistry, strong-field physics, and novel accelerators and light sources.
Figure 1 Intense single-cycle infrared pulses generation by “photon deceleration” in a plasma structure
Since the 1980s, ultrafast femtosecond lasers and their applications have become an important research topic in many frontier research fields. In the past 20 years, two Nobel Prizes have been awarded to the work related to femtosecond lasers. In 1999, Professor Ahmed H. Zewail was awarded the Nobel Prize in Chemistry for his pioneer work in using ultrafast lasers to observe photochemical reactions with femtosecond time resolution. In 2005, the Nobel Prize in Physics was awarded to femtosecond laser "light comb" technology, which enabled many fantastic refined measurements of fundamental physical quantities.
With the rapid development of femtosecond laser technology, humans’ capability to manipulate short time scales has stepped into the attosecond (~10-18 s) range, the time scale of electron quantum wave packets in atoms. In 2001, by manipulating the high-order harmonics photons generated through the interaction of a femtosecond laser with atoms, a deep ultraviolet pulse with an attosecond duration (650 attoseconds) was obtained for the first time, opening a new era of attosecond pulse generation and application. At present, the shortest attainable pulse duration (43 attoseconds) is still nearly twice as long as the unit time of natural atoms (24 attoseconds, the time it takes an electron to orbit the proton in a hydrogen atom), and the corresponding photon wavelength is in the soft X-ray band. How to obtain attosecond pulses shorter than 24as, and how to further decrease the wavelength of attosecond pulses to the hard X-ray band, are two critical challenges in the field of attosecond science.
However, due to the limitation imposed by high-harmonic generation, using existing near-infrared (0.8-3.9μm) femtosecond lasers has broken through this technical bottleneck. In principle, a longer wavelength (5-10 μm) in a near-single-cycle laser is needed to effectively achieve shorter attosecond pulses and shorter photon wavelengths. Theory and simulation show that a near-single-cycle laser of 9 μm wavelength can even produce pulses of less than a single attosecond. That is to say, it is possible to push the controllable time scale into the range of zeptoseconds (~10-21 s). However, the traditional crystal-based nonlinear optics approach has encountered an insurmountable challenge in producing femtosecond near-single-cycle lasers with wavelengths longer than 5 μm. Due to the lack of broadband non-linear optical crystals, the wavelengths of near-single-single mid-infrared lasers have been limited to less than 4 μm for a long period of time. This limitation is also a bottleneck in the development of attosecond science towards shorter pulses and higher photon energy.
At the same time, the development of relativistically intense femtosecond laser technology and its applications have made great strides in the past decade, with one of its most promising applications as a laser wakefield accelerator with ultra-high acceleration capability. Large scale accelerators such as colliders for particle physics and high brightness light sources are crucial research tools for modern scientific research, and they are typically complex, expensive and large (kilometer scale). Laser wakefield accelerators have the potential to reduce these large accelerators to an ordinary laboratory and even desktop scale due to their huge acceleration gradient, thousands of times higher than that of traditional accelerators. It is in general believed that the maturing of laser wakefield accelerators will bring revolutionary changes to the application of lasers and accelerators in industry, scientific research and medical fields.
Interestingly, in addition to a "charged particle accelerator", a laser wakefield is also a "photon decelerator" for the laser pulse. The drive laser loses its energy to produce wakes, while at the same time the frequency of the laser photons decreases (the wavelength increases), and its equivalent velocity (group velocity) in the plasma slows down ("photon deceleration"). From another perspective, this process is a nonlinear frequency conversion process of ultra-intense laser in plasma, an effect very similar to optical self-phase modulation in common crystals or gases. The key difference is that plasma is a highly flexible and customizable medium, and it can easily support ultra-intense light fields. Therefore, "photon deceleration" in a specially designed plasma structure has great potential in producing long-wavelength intense laser pulses. Finding a suitable structure to solve the longstanding challenge of generating near-single-cycle intense mid-infrared laser pulses with a wavelength longer than 5μm becomes a meaningful research area to explore. With this goal in mind, Professor Wei Lu and Associate Professor Chih-Hao Pai have advised PhD student Zan Nie to conduct in-depth analysis and large-scale particle-in-cell simulations in this field, and found a "sandwich" plasma structure that can satisfy the goal of efficiently generating intense near-single-cycle infrared pulses in the range of 5-14 μm (Fig. 2).
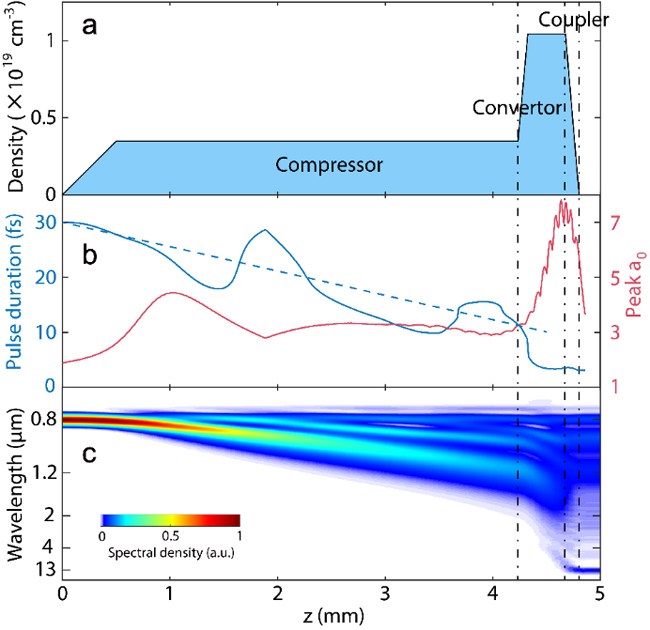
Figure 2 The “sandwich” plasma structure (a) and the generated intense single-cycle infrared pulse (central wavelength 12 μm) and its spectrum (d)
Professor Wei Lu and Associate Professor Chih-Hao Pai are the corresponding authors, and Zan Nie (PhD candidate of 2012) is the first author. This work was supported by the National Natural Science Foundation of China and the National Basic Research Program of China. The simulations were performed on Sunway TaihuLight.
Since 2011, Professor Wei Lu has been a full Professor in the Department of Engineering Physics of Tsinghua University, where he dedicated his energy to building a leading world team in laser plasma physics and advanced accelerator technology. He was awarded the first John Dawson Prize in the field of laser plasma acceleration in 2007, and the IUPAP (International Union of Pure and Applied Physics) Young Scientists Award in 2014.
The paper link:
https://www.nature.com/articles/s41566-018-0190-8
The cover link:
http://www.nature.com/nphoton/